The go-to methods for researchers and pharmaceutical companies often boil down to two primary avenues: using two-dimensional (2D) cell cultures for studies within lab settings (in vitro) and relying on animal models for live experiments (in vivo).
But here is the catch: while these methods have been the backbone of scientific exploration, they started to reveal their limitations. Both 2D cultures and animal testing, despite their widespread use, fall short in giving a complete understanding of the intricate molecular mechanisms driving various diseases. They are not reliable enough for accurately predicting how new therapies and drugs might perform in real-world scenarios before they reach clinical trials.
On one side, 2D cell cultures have been the bedrock of scientific exploration, offering reliability, affordability, and reproducibility. However, their simplicity is proving to be a double-edged sword in a scientific landscape that craves models mirroring real physiological conditions. While these cultures serve as a starting point, their findings are now seen as preliminary, especially in drug efficacy and toxicity testing. Placing cells on flat surfaces significantly alters their behaviour, affecting essential functions like growth, differentiation, and response to stimuli.
The missing piece?
The physiological complexity and dynamic interactions between cells and their environment—absent in these flat culture dishes. In essence, these cultures, while useful, offer only a fraction of the big picture. They lack the richness and depth needed to truly understand the complexities of living systems, urging us to explore more advanced models to propel our scientific understanding forward.
On the other side of the spectrum, animal models stand as a comprehensive and systemic approach to understanding molecular pathways and drug responses, offering an alternative landing for research. Yet, this approach is not without its drawbacks. Ethical considerations, high maintenance costs, and, notably, species-specific differences all contribute to their limitations in accurately predicting outcomes, especially in the field of pharmacology. Despite being the standard in preclinical studies, animal models often falter. An astounding 80% of therapies showing promise and safety within these models fail when translated to human clinical trials. This striking discrepancy underscores the challenge of extrapolating findings from animals to humans, prompting a pressing need for more reliable predictive models that can better simulate human complexities, ultimately enhancing the translational success of therapies from bench to bedside.
Substantially, these traditional models are like puzzle pieces that do not quite fit together. They’re missing the full picture, leaving gaps in our knowledge and making it harder to develop effective treatments.
So, what is next?
In recent years, a quest to merge the best aspects of traditional models has given rise to three-dimensional (3D) cell cultures, marking a significant stride in creating more lifelike and relevant experimental models. These 3D cultures step beyond the constraints of traditional methods, offering a closer emulation of the complexities found in living organisms. They achieve this by fostering a more realistic extracellular environment, a crucial element in designing models that mirror the intricacies of biological systems.
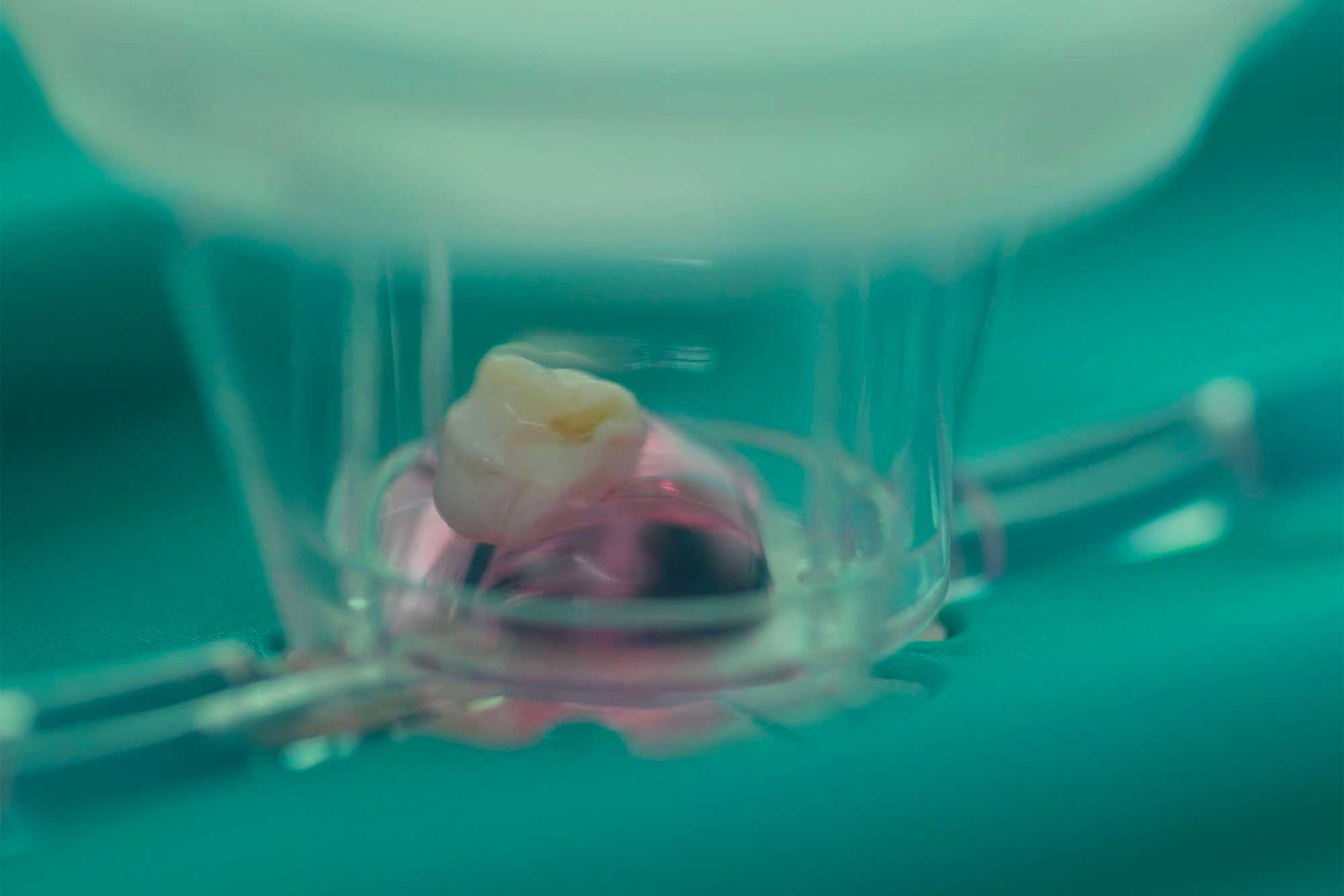
3D cultures hold immense promise in cancer research, where they enable the replication of a tumor microenvironment (TME) that closely resembles in vivo conditions while retaining the simplicity and adaptability of in vitro cell cultures. This unique versatility serves as a vital bridge between the complexities of real biological systems and the convenience of established laboratory protocols.
Understanding the tumor microenvironment’s pivotal role in tumor progression, immune evasion, and metastasis is paramount. 3D cultures allow researchers to tailor the extracellular matrix (ECM) based on specific cell types, offering a remarkable advantage in studying intricate cellular interactions and crosstalks within a more physiological microenvironment.
In labs worldwide, a fascinating array of 3D models has gained immense popularity among researchers. These models offer a more realistic portrayal of biological systems and include:
- Spheroids: Self-assembled 3D cultures mimicking tumor growth, providing insights into cancer behavior and response to treatments.
- Organoids: Structured and differentiated cellular clusters, replicating the architecture and function of organs and serving as miniaturized organ models.
- Polymeric scaffolds: Porous structures supporting cell growth, creating complex tissue-like models for detailed research.
- Reconstructed tissues: Tissue models obtained through 3D printing or cell differentiation, resembling natural structures and allowing precise experimentation.
- Patient-derived biopsies: Cells extracted directly from patients, offering a personalized and disease-specific model for tailored investigations.
The adoption of these 3D cultures represents a significant leap forward in replicating cellular behavior more similar to real physiological responses observed in living organisms. These models better mimic cell functions such as growth, gene and protein expression, migration, and drug responses, providing a closer representation of how cells behave within our bodies. This nuanced approach is invaluable, offering deeper insights into disease mechanisms and potential therapeutic interventions.
Introducing fluid-dynamic stimuli to 3D cell cultures has revolutionized in vitro models, allowing for a deeper exploration of cellular behaviors like migration, infiltration, cancer metastasis, cancer-immune cells crosstalk, and providing a more reliable platform for testing novel therapies and drugs. The dynamic environment in which cells are located is more comparable to in vivo conditions, also creating a fluid shear stress, which induces organ polarity. Zooming into the microscale, the fluid acts primarily as a laminar flow, which enables individual streams of fluid to flow separately without physical barriers. This results in a stable gradient of biochemical molecules, which physiologically regulates several biological phenomena, including angiogenesis, invasion, and migration.
MIVO® technology
Our patented MIVO® technology embodies this advancement, offering a reliable fluidic platform. It features an optically transparent cell culture chamber specifically designed to host standard 24-well plate inserts. By connecting the MIVO® device to a peristaltic pump, we replicate the systemic circulation in vitro, cultivating clinically relevant-sized cancer tissues and patient-derived models under meticulously controlled physiological flow conditions.
What’s remarkable is that these interconnected chambers pave the way for a multi-organ platform. This flexibility allows for a wide range of studies, from mirroring cancer cell journeys to metastatic sites to simulating immune cell behaviour in a lifelike environment. It also enables the exploration of new drug absorption, distribution, metabolism, and excretion across various tissues concurrently—a breakthrough in achieving more accurate and holistic in vitro studies.
This pioneering approach revolutionizes how we conduct diverse research, offering a more realistic and controlled environment to explore complex biological processes and potential therapeutic interventions.
Innovative fluidic platforms like MIVO® technology herald a new era in biomedical research, unlocking the secrets of cellular behaviour within a controlled in vitro environment—bridging the gap between lab studies and real-world physiological complexities.
To learn more about MIVO® Platform click here.